Thin-walled sleeve parts are challenging to machine because they deform under cutting forces, thermal effects, and vibrations. One critical factor contributing to this deformation is the initial residual stress, which occurs due to external forces or uneven temperature in the manufacturing process, leading to uneven elastic-plastic deformation. Processing analysis and successfully applying these parts involve understanding the material behavior, optimizing machining strategies, and leveraging precision control techniques.
I. Characteristics of Thin-Walled Sleeve Parts
Thin-walled sleeves have low radial stiffness, making them prone to deformation under cutting forces. Their high surface area-to-volume ratio leads to heat buildup during machining, causing thermal expansion. These parts are sensitive to vibrations, resulting in chatter and poor surface finish. Residual stresses from prior processes can cause unexpected deformations during machining. Clamping these delicate components without distortion is challenging, requiring specialized techniques. Finally, they demand high precision, with strict tolerances for dimensions, roundness, and coaxiality.
II. Challenges in Machining Thin-Walled Sleeve Parts
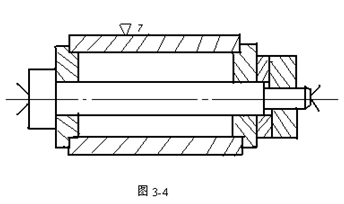
1. Machining Deformation Under Cutting Forces
The elastic deformation and weak radial stiffness of thin-walled sleeve components lead to significant machining deformation during cutting. This affects the machining accuracy of the outer circle, inner hole, and overall wall thickness, making it challenging to maintain critical tolerances like roundness and coaxiality.
2. Thermal Expansion and Poor Heat Dissipation:
During machining, the heat generated, especially with high-speed cutting and aluminum alloys, leads to cutting heat buildup, causing machined surface distortion. Pour sufficient cutting fluid during machining processes to reduce the workpiece’s thermal deformation. Poor heat dissipation further aggravates thermal expansion, leading to residual stress redistribution and impacting surface quality. Sufficient cutting fluid and optimizing the cutting-edge angle can help mitigate these effects.
3. Vibrations and Chatter:
Due to their low rigidity, thin-walled parts are highly susceptible to vibrations. These vibrations can lead to chatter, reducing machining quality and increasing tool wear on the turning tool and milling cutter. Proper clamping position and support with front and rear centers can help control these vibrations.
4. Clamping and Fixturing:
The clamping position of thin-walled parts requires careful attention to prevent workpiece deformation. Uneven forces can lead to waist shape or ovalization of the part, affecting both the inner circle and outer wall dimensions. Innovative fixturing, such as elastic mandrels, is essential for minimizing clamping deformation while improving machining quality.
5. Residual Stresses and Stress Redistribution:
Initial residual stresses from prior processes, like heat treatment, can cause machining errors as these stresses are released during material removal. The stress distribution changes when using rough turning tools, leading to residual stress redistribution, which can deform the thin-walled casing. Optimizing process parameters and tool paths can mitigate these issues.
6. Material Removal and Machining Efficiency:
Machining parameters such as cutting speed, feed rate, and cutting margin are crucial in balancing material removal and part stability. High material removal rates lead to excessive deformation, while slow rates reduce machining efficiency. Ensuring that the rough turning tool requires minimal cutting forces helps prevent excess stress on the part while maintaining machining quality.
7. Achieving Tight Tolerances and Surface Quality:
Thin-walled parts demand high machining accuracy and surface roughness control, especially in fine-turning. Maintaining hole quality inspection standards for inner holes and ensuring that the outer turning tool delivers precision cuts are essential. Using a coordinate measuring machine (CMM) to verify tolerances ensures processing quality and dimensional accuracy.
8. Chip Removal and Tool Wear:
Efficient chip removal prevents heat buildup and surface damage. Pour sufficient cutting fluid to improve processing quality by correctly evacuating chips and reducing tool wear. Cutting outflow direction optimization and high-pressure sufficient cutting fluid enhances processing quality by correctly evacuating chips, reducing tool wear, and improving machining efficiency.
III. Cutting-Edge CNC Machining for Thin-Walled Parts
Advanced CNC machining techniques significantly improve the accuracy and precision of thin-walled sleeve parts. High-speed machining reduces machining time and enhances precision by minimizing heat buildup and deformation. High-precision machining ensures that even the most intricate features are accurately produced, while micro-machining allows for creating small, detailed features with exceptional accuracy. Milling is particularly effective for machining complex geometries, and turning is ideal for cylindrical parts, achieving high precision and surface quality. These cutting-edge techniques, combined with optimized machining parameters and real-time monitoring, enable the production of high-quality thin-walled sleeve components, meeting the stringent demands of modern industries.
IV. Techniques of CNC Turning for Thin-Walled Sleeve Parts
Machining thin-walled sleeve components require precise control to avoid deformation and ensure surface quality. Key techniques include:
Low-Force Machining: Optimized cutting parameters reduce cutting forces, minimizing deformation and improving machined surface quality.
Optimized Clamping: Using elastic mandrels or vacuum chucks prevents workpiece deformation, distributing forces evenly.
Step-by-Step Machining: Begin with rough turning and finish with fine tuning to control residual stresses and ensure accuracy.
Cooling: Sufficient cutting fluid reduces cutting heat and enhances tool life.
Monitoring: Real-time monitoring with a coordinated measuring machine ensures consistent machining quality.
V. Cutting-Edge CNC Cutting Process for Thin-Walled Parts
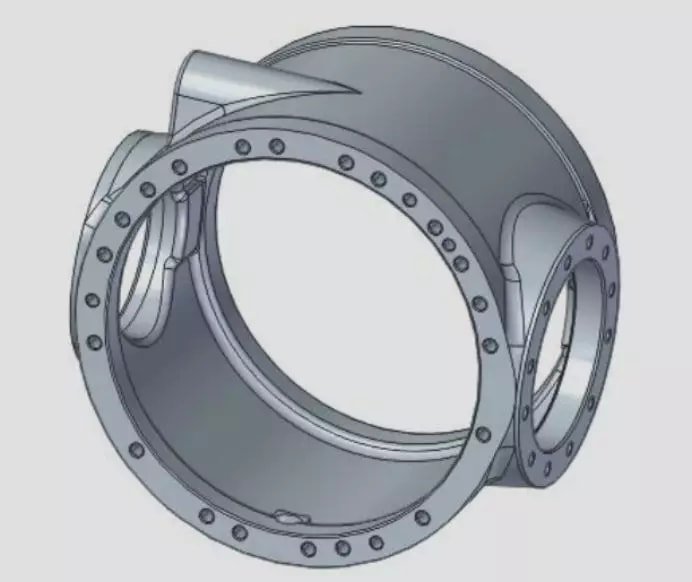
Advancements in CNC machining enable efficient and accurate processing of thin-walled parts. The machine tool spindle is crucial in advanced machining systems, particularly in experiments involving dynamic characteristics and modal analysis. Modern CNC systems incorporate several features that improve the machining process, such as:
High-Speed Machining (HSM):
Increasing cutting speeds reduces cutting heat by allowing chips to carry heat away, minimizing thermal deformation. This is particularly beneficial for materials like aluminum alloys and thin-walled sleeve components, ensuring precise dimensions while avoiding machining deformation.
Adaptive Control:
CNC machines with real-time feedback systems adjust feed rates and tool paths based on cutting forces during the cutting process. This helps maintain machining accuracy by minimizing part distortion, even when processing thin walls with low radial stiffness.
Tool Wear Compensation:
Modern CNC systems can automatically adjust for tool wear, ensuring continuous accuracy in long production runs. The machine tool spindle plays a crucial role in ensuring continuous accuracy and maintaining machining quality. It maintains machining quality by accounting for tool wear during high-speed cutting, optimizing the cutting-edge angle, and providing consistent machined surface quality.
Combined with optimized machining parameters, clamping positions, and sufficient cutting fluid, these innovations improve thin-walled components’ surface quality and machining efficiency, reducing residual stress and improving machining quality in mass-production scenarios.
VI. Process Analysis in Thin-Walled Part Machining
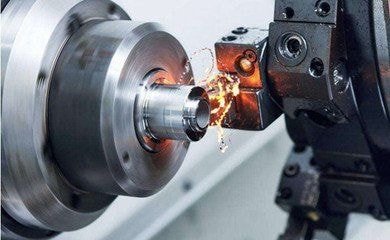
Accurate machining process analysis is crucial for controlling cutting forces, generating heat, and ensuring workpiece stability when machining thin-walled parts. Initial residual stress, caused by external forces or uneven temperature during manufacturing, plays a significant role in machining deformation. Key factors include:
Finite Element Analysis (FEA): This simulation technique allows engineers to predict how thin-walled components react to different cutting conditions, such as cutting force and residual stress distribution. It helps identify areas prone to machining deformation and optimize machining strategies for improved machining quality.
Thermal Control: The heat generated during the cutting process can distort the thin walls of a component, especially when machining aluminum alloys or thin-walled casings. Using sufficient cutting fluid and optimizing cutting speed and edge angle helps manage heat dissipation, minimizing thermal deformation and improving machined surface quality.
Cutting Force Decomposition: The three components of cutting force—Fz (main cutting force), Fx (feed resistance), and Fy (cutting resistance)—must be carefully analyzed. Understanding their impact on elastic deformation and stress distribution provides insights into minimizing machining errors and enhancing machining accuracy.
Combined with optimized tool paths, turning tools (e.g., rough turning tool, outer turning tool), and sufficient cooling, these factors contribute to effective machining processes for thin-walled sleeve components, improving processing quality and reducing residual stresses.
VII. Key Technologies for Machining Holes in Thin-Walled Parts
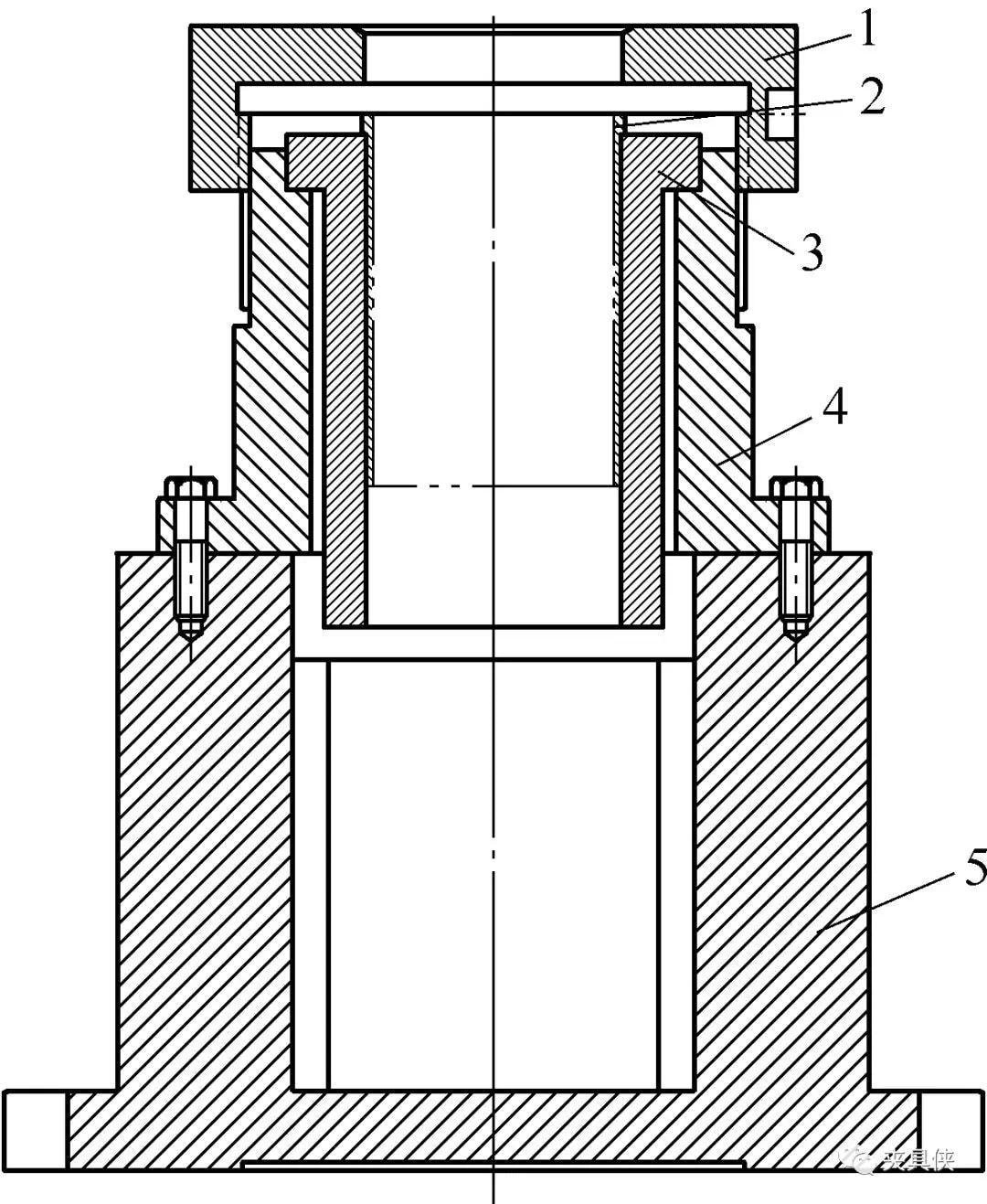
Machining holes, particularly in thin-walled parts, requires advanced techniques to ensure precision. Key technologies include:
Boring and reaming operations are critical for achieving high-precision inner diameters and maintaining coaxiality between surfaces.
Use of damping tools: Specialized tools that reduce vibrations ensure accuracy when machining delicate internal features.
Coolant delivery systems: Effective coolant systems help evacuate chips and maintain part stability by reducing thermal buildup during hole machining.
VIII. Solving the Problem of Chip Removal in Thin-Walled Part Machining
1. High-Pressure Coolant Systems: These systems improve the cutting process by efficiently removing chips from the cutting zone, which prevents chip buildup that could negatively affect machined surface quality and cause machining errors. Additionally, the coolant helps reduce cutting heat, especially in the milling process of aluminum alloys and thin-walled sleeve components.
2. Optimized Tool Geometry: Tools with advanced cutting-edge designs, such as specialized chip-breaking geometries, ensure small, manageable chips are produced. This minimizes the part’s stress distribution and enhances the machining process’s efficiency for thin-walled parts.
3. CNC-Controlled Pecking Cycles: For deep-hole machining, pecking cycles allow the hole-turning tool to retract periodically, clearing chips from the hole. This enhances chip removal, protects the machined surface from damage, and maintains machining quality.
These strategies help overcome challenges related to poor heat dissipation and chip evacuation, ensuring machining accuracy, especially in high-speed or mass-production scenarios involving thin walls.
IX. Processing Methods for Thin-Walled Parts
1. Axial Clamping: This technique applies force along the axis of the workpiece to avoid radial deformation and maintain part stability during the cutting process. By optimizing the clamping position, deformation is minimized, which is essential for thin-walled sleeve components.
2. Step-by-Step Machining: Alternating between rough and fine turning stages ensures gradual material removal. Rough turning tools are used for bulk material removal, while outer turning tools are employed during finishing stages to achieve the desired surface quality and reduce residual stresses.
3. Optimized Feed and Speed: Lower feed rates and higher cutting speeds minimize cutting forces, reducing the risk of elastic deformation or chatter. This method is particularly effective in high-speed cutting of aluminum alloys or thin-walled casing components, improving machining efficiency and preventing errors.
Conclusion
Machining thin-walled parts will continue to improve with advancements in CNC technology, adaptive control, and real-time monitoring. Future developments in tool materials, chip removal systems, and cooling techniques will enhance precision and machining quality. As automated quality control and AI-driven processes become more prevalent, manufacturers can expect better accuracy and efficiency, meeting the increasing demand for lightweight, high-performance components.